February 2022 Issue
Researcher Video Profiles
Kali Prasanna Nayak
Associate Professor, Department of Engineering Science
Developing a single atom quantum interface on a tapered optical fiber
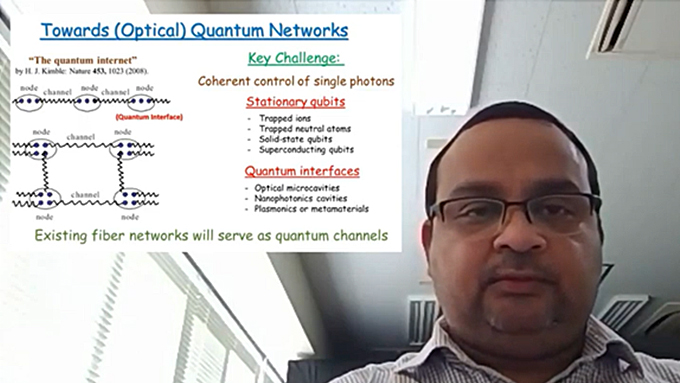
In this video, Kali Prasanna Nayak describes his research on developing a single atom quantum interface on a tapered optical fiber, starting with an overview of the concept of quantum interfaces and recent developments in quantum computers based on trapped ions, trapped neutral atoms or superconducting qubits. Nayak says that the “next challenge will be how to connect these quantum nodes over a network where single photons will be the information careers; the so-called quantum internet.”
Notably, single photons can be efficient carriers of quantum information because they can move at the speed of light and do not easily interact with the surrounding medium. However, these same properties lead to difficulties in isolating and controlling single photons. One way to resolve this problem is the development of quantum interface platforms where single photons can be controlled/manipulated using an ensemble of atoms.
“If this could be done at the single atom level then one can use the quantum properties of the single atom to further enhance the quantum functionality of such a quantum node,” says Nayak.
But the question is why is it so difficult to realize a single atom quantum interface?
One way of overcoming this problem can be devised by considering the efficiency with which a photon interacts with a single atom. To quantify this, the parameter known as the ‘single atom cooperativity’ is defined that is the ratio of single atom absorption cross-section to the photonic mode area.
“This means that we have to strongly confine the single atoms and photons to subwavelength dimensions to realize efficient interaction,” explains Nayak. “This is about transverse confinement. Another way is to confine a single atom inside a high finesse optical resonator, where the interaction will be further enhanced by the number of photon round-trips inside the cavity i.e. the finesse of the cavity. This is called longitudinal confinement.”
These ideas are being pursued worldwide to develop a quantum interface using micro-cavities and nanostructures. “In my group, we are trying to develop a quantum interface on an optical nanofiber i.e. a tapered optical fiber with subwavelength diameter waist,” says Nayak. “The diameter of the nanofiber is almost half of wavelength, with the primary advantage of such a technique is that the fiber guided mode can be strongly confined in the transverse direction to subwavelength dimensions while interacting with the surrounding medium in the evanescent region. Apart from the transverse confinement, one can also introduce an in-line cavity on the nanofiber to further enhance the atom-photon interactions. After all this is a fiber in-line technology easily integrable to fiber networks.”
Nayak also describes the advantage of transverse confinement, where he compares a free-space cavity with a nanofiber cavity, stressing that to realize a cooperativity of ten in free-space cavities requires a finesse of half a million. Similar cooperativities can be realized on a nanofiber cavity with a moderate finesse of hundred to two hundred. This is because of the strong transverse confinement in nanofiber cavity. In free-space cavities, the effective mode radius is order of tens of microns whereas that for a nanofiber cavity is only half a micron; this is the key advantage.
Cavities are fabricated in thin fibers using femtosecond laser fabrication techniques to produce photonic crystal cavities on nanofibers.
The next question is how to trap a single atom on such a nanofiber cavity? “We use this kind of tightly focused optical dipole trap, the so-called single atom tweezer, to trap individual atoms on the nanofiber cavity,” says Nayak. “The key point of such a tightly focused trap is that the trap volume is so small that it can trap only one atom at a time. If two atoms enter the trap, they will collide with each other and will be lost from the trap. It is known as the collisional blockade mechanism.”
Nayak and his group have conducted wide variety of experiments based on this approach and have produced a nanofiber cavity and an optical tweezer beam tightly focused on the nanofiber. “We load atoms into the nanofiber trap from a laser-cooled atom cloud formed by a standard magneto-optical trap (MOT) and monitor the photon counts through the fiber guided modes. We observed the photon count rates as a function of time and saw clear single-step-like signals. This indicates that single atoms are trapped and interfaced to the nanofiber. The fluorescence from the trapped single atom is coupled to the nanofiber guided modes and that results in such step-like fluorescence signals.”
Nayak and his group confirmed these results by measuring the photon correlations, where anti-bunching of fluorescence photons clarified that these signals were from individual single atoms. “We have also measured the trap lifetime to be around 50 ms, and from photon counts we estimated a cooperativity of 5.4 and channeling efficiency as high as 85%,” says Nayak. “This means that almost 85% of the atomic emission is coupled into the fiber guided modes. That’s a very efficient single atom quantum interface. Such interfaces offer new possibilities for fiber in-line quantum nodes and single photon manipulation.”
Research Keywords: Quantum Optics, Nanophotonics, Nanofiber optics, Laser cooling and trapping, Control and manipulation of single atoms and single photons